Abstract
Discover the groundbreaking shift in global energy and industry brought about by Direct Lithium Extraction (DLE). This comprehensive article delves into how DLE is redefining the lithium supply landscape, offering a more sustainable and efficient pathway to meet the soaring global demand for lithium. Uncover the transformative effects of DLE on energy storage solutions, the electric vehicle market, and renewable energy systems. Explore in-depth analyses, including Goldman Sachs’ comparative scenario projections, and understand DLE’s role in shaping a low-carbon future. Dive into case studies, technological innovations, and the economic implications of this cutting-edge technology. Whether you’re an industry professional, a sustainability enthusiast, or simply curious about the future of energy, this article offers valuable insights into the pivotal role of lithium in next-generation energy technologies.
Introduction
The dawn of the Industrial Revolution witnessed the transformation of societies. Driven by coal combustion, economic paradigms shifted as powering steam engines enabled mass production, catalyzing trade and commerce on an unprecedented scale. As the wheels of industry turned, they ushered in an era of innovation and productivity that forever altered the trajectory of human civilization. Energy transitioned from the depths of coal mines to the vast expanses of oil fields.
Hydrocarbon combustion became a fundamental driver of the 20th century’s technological and industrial expansion. From the widespread use of petroleum in automobiles to natural gas heating our homes, these resources have become the lifeblood of modern society.
However, these traditional methods of energy sourcing have raised environmental concerns due to their invasive nature and costly carbon footprint. Renewable energy alternatives have emerged as a promising solution to address these challenges. Central to this discourse is our ever-growing electrical demand, driven by an unprecedented pace of technological innovation.
The transformation from a hydrocarbon-based economy to an increasingly electric and renewable economy has highlighted lithium as a pivotal element in this global transition. Lithium has become thev bedrock of energy storage solutions; lithium-ion batteries are crucial for the widespread adoption of electric vehicles (EVs), portable electronics, and renewable energy storage systems.
Overall supply and demand of lithium for batteries by sector, 2016-2022
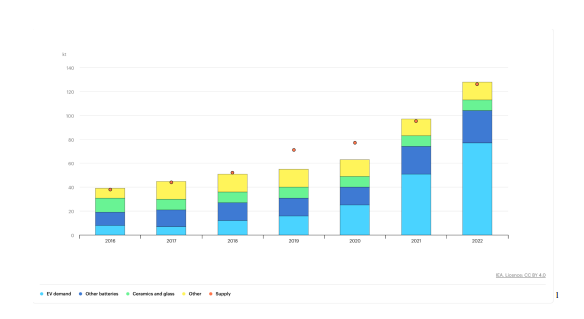
Current trends indicate a robust increase in the demand for lithium, largely propelled by the automotive sector’s shift towards EVs. The growing market for personal electronic devices also contributes to this heightened demand. This surge is prompting a re-evaluation of lithium supply chains, which traditionally have relied on both time-consuming and environmentally taxing mineral and brine extraction processes. In the face of these demands, the industry is looking at the forecasted supply capabilities and realizing the necessity for enhanced lithium recovery methods.
Direct Lithium Extraction (DLE) technologies are at the forefront of this innovation, poised to offer a transformative solution. DLE can expedite lithium production with a significantly smaller environmental footprint. DLE can deliver a much faster turnaround from extraction to market by circumventing the lengthy evaporation processes associated with traditional extraction methods.
The challenge, however, lies in scaling these technologies to meet global demands. As we approach the third decade of the 21st century, additional lithium sources are expected to be delivered to market froM conventional early-stage mineral and brine projects and less conventional sources like geothermal or oilfield brines. The integration of these new supply streams, augmented by innovative extraction technologies like DLE, aims to address both the immediate and long-term requirements of the lithium market.
IEA, Overall supply and demand of lithium for batteries by sector, 2016-2022, IEA, Paris https://www.iea.org/data-and-statistics/charts/overall-supply-and-demand-of-lithium-for-batteries-by-sector-2016-2022, IEA. Licence: CC BY 4.0
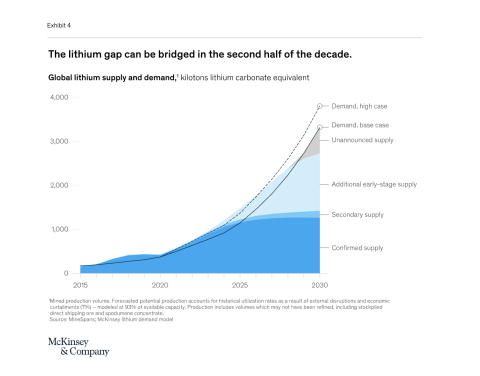
Yet, bridging the supply gap is not merely a question of increasing raw output. It involves optimizing the entire supply chain to enhance efficiency, from extraction through to the delivery of the final product. Direct Shipping Ore (DSO) initiatives, for example, can mitigate some of the immediate risks associated with undersupply by streamlining the logistics of getting lithium to market. Direct Shipping Ore (DSO) refers to a method in mining where the ore is mined and shipped directly to the customer without any additional processing or beneficiation. This approach is typically used when the ore is of a sufficiently high grade that it does not require any processing to meet the customer’s specifications. By adopting DSO Azevedo, M., Baczyńska, M., Hoffman, K., & Krauze, A. (2022, April 12). Lithium mining: How new production technologies could fuel the global EV revolution. McKinsey & Company. initiatives, companies can significantly reduce the time and cost associated with processing lithium ore.
This not only accelerates the delivery of lithium to the market but also lowers the overall cost of production. Moreover, DSO minimizes the environmental footprint of the mining process, as it bypasses the need for energy-intensive refining processes. Ultimately, implementing DSO strategies can be a key component in ensuring a more sustainable and efficient lithium supply chain, essential for meeting the burgeoning demand in various industries, particularly in producing lithium-ion batteries for electric vehicles.
The bottom line is that the lithium market’s current state is one of cautious optimism. The industry can meet the growing demand with suitable investments in technology and infrastructure and a focus on sustainability. As we continue to prioritize renewable energy and electric mobility, lithium remains a critical component of our energy future, necessitating a concerted effort to develop more sustainable and efficient methods of procurement and supply.
What is Direct Lithium Extraction (DLE)?
Direct Lithium Extraction (DLE) signifies a transformative approach to procuring lithium, crucial for the burgeoning demand from sectors like electric vehicles (EVs) and large-scale energy storage. It harnesses advanced techniques that promise to improve the efficiency and reduce the environmental footprint of lithium recovery. Each of the three primary DLE methods—adsorption, ion exchange, and solvent extraction—brings a unique set of chemical interactions and processes designed to selectively extract lithium from complex brine solutions containing various other dissolved minerals.
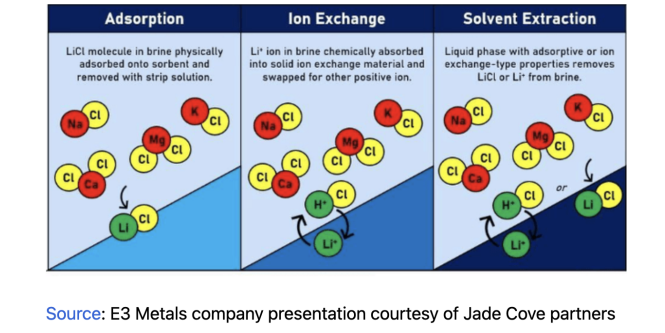
The first of these, adsorption, involves using materials with porous surfaces with a high affinity for lithium ions. These materials act somewhat like a sponge, selectively soaking up lithium ions from the brine. This selectivity is crucial, given the varying composition of lithium brines, which can include a multitude of other ions that must be excluded from the recovery process. Once the lithium ions are adsorbed onto the material, they can be stripped and collected for further refinement.
Ion exchange, the second technique, is a chemical process where lithium ions in the brine are swapped with other ions (like hydrogen or sodium) attached to an ion exchange material. This swapping is facilitated by the specific chemical properties of the ion exchange material, which is designed to bind with lithium ions preferentially. After the exchange, the material is treated to release the lithium ions, which are then concentrated and extracted as lithium compounds.
The third technique, solvent extraction, involves using a solvent that can selectively dissolve lithium ions from the brine. This method relies on the solvent’s chemical composition. The solvent is chosen depending on its ability to react with and extract lithium ions. After the solvent has absorbed the lithium ions, it is separated from the rest of the brine. Then, the lithium is extracted from the solvent through further chemical processes. Scaling these DLE technologies from laboratory to industrial production poses numerous challenges, primarily related to the efficiency and durability of the materials and processes involved. The intricacy of the brine’s chemical composition requires highly selective and robust extraction media, whether solid adsorbents, ion exchange resins, or solvents. The future of lithium extraction will likely depend on the continued development and refinement of these DLE technologies, as they promise to meet the high demand for lithium with a minimal environmental footprint.
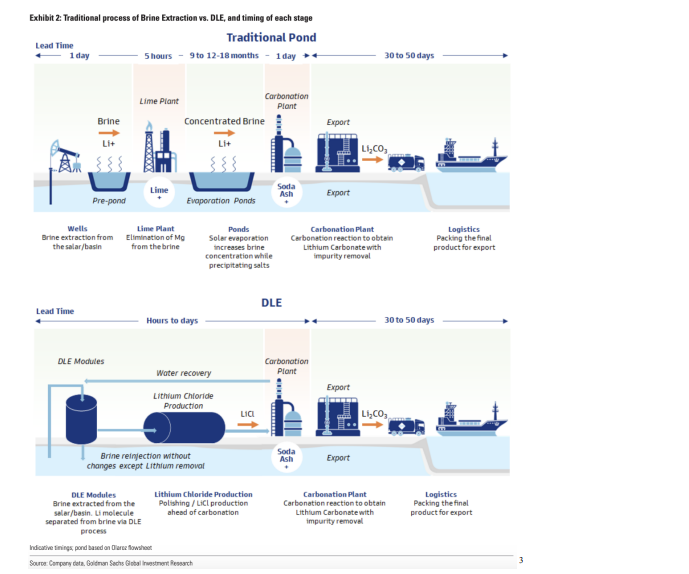
It is much easier to visualize comparing the traditional brine extraction process for lithium with the more modern DLE technique. The top half of the image details the traditional method, which is a time-intensive sequence starting from brine extraction from wells. The extracted brine, rich in lithium, is then transferred to ponds where natural solar evaporation occurs over 9 to 12-18 months. During this time, the lithium concentration increases as water evaporates. Additives like lime are used to remove impurities such as magnesium. The concentrated brine goes through a carbonation process to precipitate lithium carbonate, which is harvested and prepared for export.
The bottom half outlines the DLE process, significantly reducing the lead time from months to hours or days. DLE modules extract lithium directly from the brine without needing expansive evaporation ponds, rapidly producing lithium chloride. The process includes steps for water recovery and lithium chloride polishing before moving to carbonation, where lithium carbonate is produced. This direct method is quicker and more environmentally considerate, allowing for the reinjection of the brine into the original source with minimal alterations primarily, the extraction of lithium. This reduces the environmental footprint by eliminating the need for large evaporation ponds and reducing land and water use. The DLE process concludes with the packaging and export of lithium carbonate, paralleling the final steps of the traditional method but achieving them more efficiently and sustainably.
Case Study: E3 Metal Corp
DLE technologies represent a significant shift from conventional lithium production, focusing on improved efficiency and environmental sustainability. In the DLE industry, companies often develop their own proprietary technologies and processes; E3 Lithium is no exception. Their specific DLE method is crafted to enhance lithium recovery and address environmental and economic challenges. This tailored approach positions E3 Metals as a case study in the application and potential of custom DLE solutions. E3 Lithium has developed a DLE process for their Clearwater Project in Alberta, Canada, which is designed to optimize lithium recovery from the Leduc Aquifer. Their resources total 7.0 million tonnes of
contained Lithium Carbonate Equivalent (LCE) with notable lithium grades. E3’s technology is engineered to achieve over 90% lithium recovery, a substantial increase compared to traditional extraction methods. This high recovery rate is complemented by the process’s ability to significantly concentrate lithium while removing over 99% of impurities, including common contaminants like calcium, magnesium, and sodium
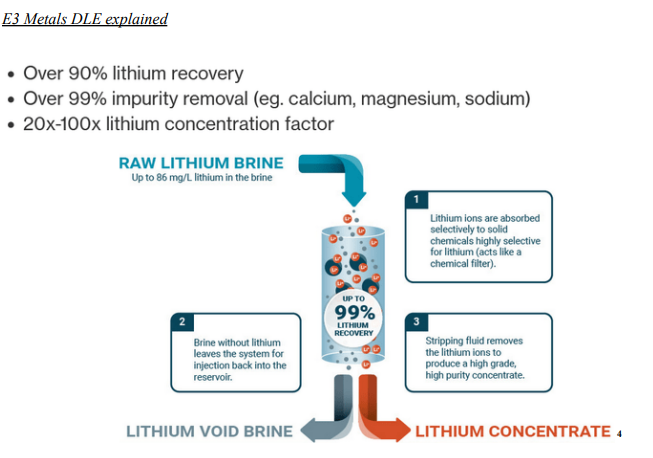
The company’s DLE technique leverages ion exchange technology using a proprietary sorbent. This sorbent is highly selective for lithium ions, enabling the process to efficiently filter large volumes of low-grade brine and produce a high-grade lithium concentrate.
4 E3 Metals Corp. (n.d.). Our technology. E3 Lithium. Retrieved from https://www.e3lithium.ca/our-assets/technology
Summary of the Clearwater Project PEA economics
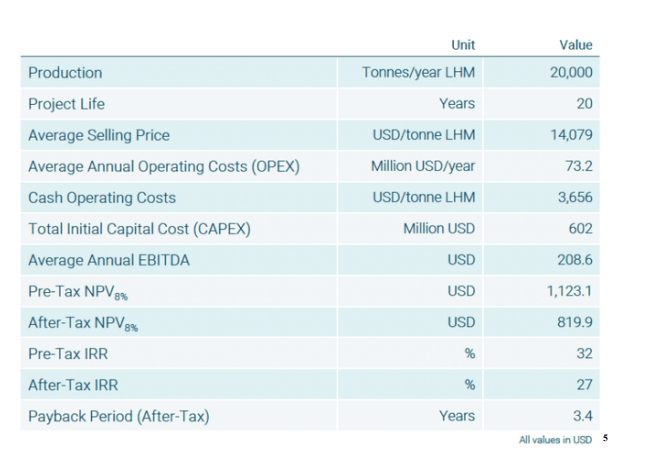
From an economic perspective, the Clearwater Project’s Preliminary Economic Assessment (PEA) demonstrates promising figures, with a post-tax Net Present Value (NPV%) of US$819.9 million and an Internal Rate of Return (IRR) of 27%. The estimated initial capital cost is US$602 million, with operational expenses calculated at US$3,656 per tonne of lithium hydroxide monohydrate (LHM). These figures suggest a robust economic case for the project, which is now progressing towards the pilot testing stage, aiming to achieve a production target of over 20,000 tonnes of lithium hydroxide per annum by 2025-26.
The case of E3 Lithium serves as a testament to DLE technologies’ viability and future potential in meeting the growing demand for lithium, necessary for the EV market and renewable energy storage solutions. As such technologies advance, they are set to play a pivotal role in the global shift towards sustainable energy practices
5 E3 Metals Corp. (n.d.). Our technology. E3 Lithium. Retrieved from https://www.e3lithium.ca/our assets/technology/
Goldman Sachs’ Comparative Scenario Analysis
The full report can be accessed for a comprehensive understanding of the DLE technology’s impact on the lithium industry’s future: Goldman Sachs Direct Lithium Extraction Report
DLE is poised to be a game-changer in the lithium sector, presenting a significant advancement in how lithium is extracted from brine resources. This technology, which can dramatically cut down production times to mere hours or days and boost lithium recovery rates from 40-60% to upwards of 90%, stands to revolutionize the industry. It aligns perfectly with the current push for sustainability, as it slashes land usage by more than 20 times and presents the potential for brine reinjection, minimizing environmental disruption. Financially, DLE’s higher initial investment in cutting-edge technology is offset by substantial operational cost savings and increased annual production. Capital expenditures (CapEx), which encompass the investment in infrastructure necessary to commence production, are higher for DLE
However, the operational expenses (OpEx), or the costs of running the production process, are projected to be lower, ranging from $2,800 to $3,600 per tonne of LCE. This compares favorably to the OpEx of traditional evaporation ponds, which hover around $3,300 to $4,900 per tonne of LCE. The enhanced recovery rates make the process more efficient and ensure a more favorable economic outcome. DLE projects are anticipated to exhibit an NPV of approximately $0.6 to $1.1 billion and an IRR of around 20-30%. Such figures suggest that DLE is a technical improvement over traditional methods and a financially sound investment.
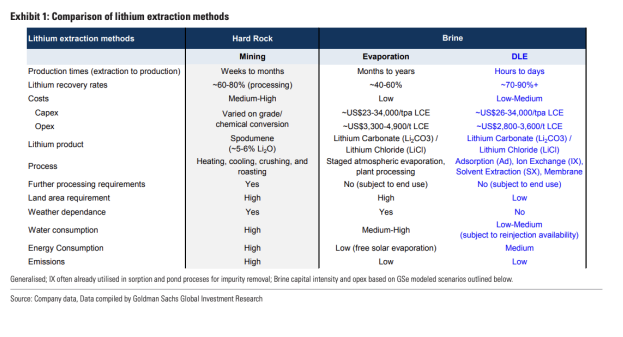
The report by Goldman Sachs underlines DLE’s potential to improve the economics of lithium extraction. It also suggests that as the technology matures and scales up, we can expect further enhancements in both CapEx and OpEx efficiencies. More importantly, companies are not merely adopting existing DLE methods but innovating and crafting their own technologies, underlining a robust competitive drive. These proprietary techniques are likely to offer unique efficiencies and cost benefits tailored to their projects’ specific mineralogical and geographical contexts. The commitment to sustainability is evident in the projected land-use reductions, highlighting DLE’s potential to deliver significant environmental benefits, such as the reinjection of brine to reduce ecological disturbance
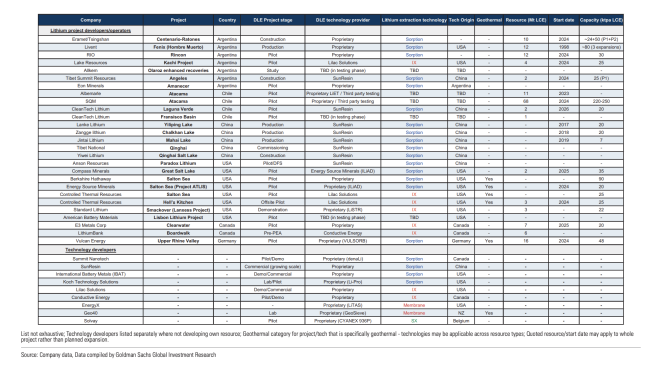
The table above illustrates the global scale of DLE initiatives, with various projects at different stages, from pilot to production. This breadth of engagement reflects a concerted industry effort toward more sustainable and economically viable lithium extraction. Building on the global scale of DLE initiatives illustrated earlier, the Goldman Sachs report delves into a comparative economic analysis of DLE versus traditional brine pond methods. This juxtaposition is theoretical and grounded in data from current operations and insights from industry experts. The analysis contemplates a hypothetical scenario where DLE and traditional methods extract lithium from a brine source with a similar grade and volume, showcasing DLE’s ability to ramp up production in significantly less time—18 months faster—despite higher initial expenses.
In terms of production, DLE’s estimated recovery rates are ambitious, aiming for 70-90% efficiency, which could yield 18-23ktpa LCE. This is a substantial improvement over the 40-60% efficiency and 10-15ktpa LCE of traditional ponds. The broader recovery range considered for DLE, from 50% to 100%, allows for a nuanced analysis that accounts for the early stage of DLE technology and its potential evolution. Investment in plant and processing for DLE is a major determinant of its higher CapEx.
However, the strategic allocation of funds towards more advanced infrastructure, as opposed to traditional ponds, is anticipated to result in long-term economic gains. These gains are characterized by the reduction in OpEx, given the more efficient recovery process, despite the upfront investment. This economic calculus underscores a shift towards prioritizing technology that, while currently more expensive, yields better returns over the lifecycle of the extraction project. Goldman Sachs’ detailed financial modeling signals a growing recognition of DLE’s value proposition, hinting at a potential industry-wide pivot to this more sustainable and viable extraction method.
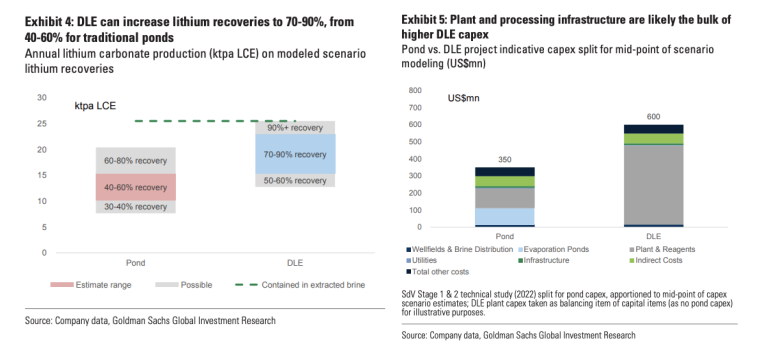
Goldman Sachs acknowledges the variability in economic outcomes for DLE projects due to differing capital (CapEx) and operational (OpEx) expenditures. The firm emphasizes that despite these variations, the pivotal aspect driving economic viability is the elevated lithium recovery rates achieved through DLE, which substantially improve annual production volumes. Goldman Sachs presents a scenario analysis contrasting DLE with conventional brine ponds, using an assumed constant production of approximately 25,000 tonnes per annum (ktpa) of Lithium Carbonate
Equivalent (LCE) over a 20-year lifespan.
The capital intensity of DLE, estimated at around $30,000 per tonne of lithium carbonate equivalent (LCE), is juxtaposed against operational costs, which are projected to be lower than traditional methods, making DLE economically competitive. Specifically, an 80% recovery DLE project requires less than $7,500 per tonne in operational costs to reach NPV breakeven, compared to less than $4,000 per tonne for a 60% recovery traditional pond, reflecting DLE’s lower operational cost per unit of lithium recovered. The breakeven OpEx decreases as the recovery rate improves, demonstrating DLE’s potential for cost-effectiveness despite higher initial investments
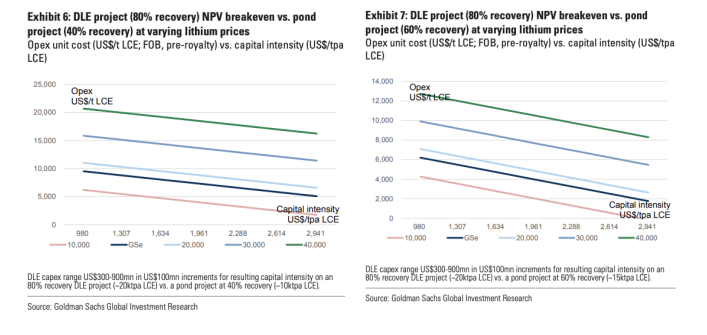
Goldman Sachs’ mid-case scenario analysis meticulously compares the projected financial performance of DLE projects against traditional brine ponds. They calculate a higher Net Present Value (NPV) for DLE projects—approximately $0.6 billion to $1.1 billion—attributed to recovery rates of 70-90%, with corresponding Internal Rates of Return (IRR) between 20-30%. In contrast, traditional brine ponds, with 40-60% recovery rates, yield lower NPVs of $0.3 billion to $0.7 billion and slightly lower IRRs of 20-25%.
The analysis also highlights that DLE’s capital intensity is on par with traditional methods after adjusting for the superior recovery rates, with estimated capital expenses of approximately $26,000 to $34,000 per tonnof lithium carbonate equivalent (LCE). These figures are based on a median upfront capital expenditure of $600 million. Despite DLE’s higher initial capital intensity compared to evaporation ponds, the anticipated lower operational costs—stemming from increased production efficiency—are expected to compensate. Specifically, DLE’s operational cost is around $2,800 to $3,600 per tonne of LCE, contrasting with $3,300 to $4,900 for traditional ponds
Economies of Scale
The journey towards a low-carbon energy system is tightly coupled with developing cost-effective energy storage solutions. Lithium-ion batteries stand at the forefront of this transformation. The past three decades have witnessed a monumental shift, with the price of lithium-ion battery cells falling by an astonishing 97%. In 1991, a kilowatt-hour (kWh) of battery capacity cost $7,500; by 2018, the price had dramatically dropped to $181. This decline demonstrates economies of scale, showing how increased production and technological improvements drive down costs.
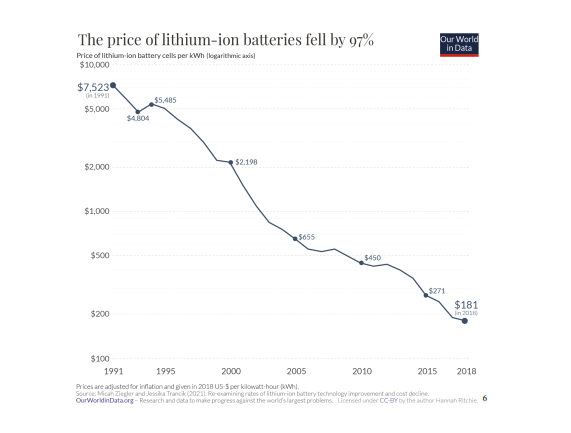
This price reduction is not merely a historical curiosity but a continuing trend, with the cost halving between 2014 and 2018. Such a rapid decrease in price is indicative of an industry scaling up and reaping Ritchie, H. (2021, June 4). The price of batteries has declined by 97% in the last three decades. Prices have historically fallen by an average of 19% for every doubling of capacity, a manifestation of Wright’s Law. This law suggests that increased production volume naturally leads to cost reductions due to efficiencies gained in manufacturing processes.
Our World in Data. Retrieved from https://ourworldindata.org/battery-price-decline the benefits of mass production.
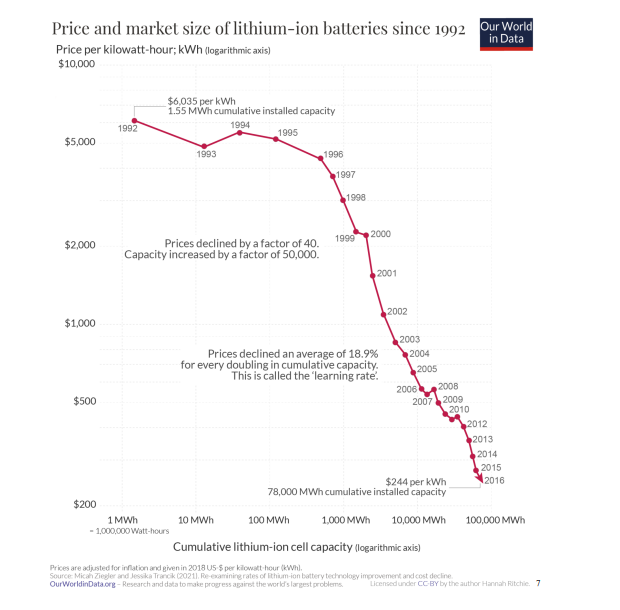
The impact of economies of scale is palpable when examining the cost of batteries in popular electric vehicles (EVs). For instance, a 40 kWh battery in a Nissan Leaf, which would cost $7,300 in 2018, would 7 Ritchie, H. (2021, June 4). The price of batteries has declined by 97% in the last three decades. Despite a year-on-year decrease in pack manufacturing costs by 5% in 2022, cell production costs saw a slight increase due to the rising prices of materials and electricity. This fluctuation emphasizes material price trends in the long-term cost trajectory of battery production.
The price reductions we observe are driven by more than just the passage of time; they are the result of relentless innovation in battery production. As production scales, technological advancements emerge, enabling the production of batteries at lower costs. This is further elucidated by examining the relationship between prices and the cumulative installed capacity of batteries. Since the market’s infancy in 1991, there has been a meteoric increase in deployed capacity, leading to a significant reduction in costs as per the learning curve.
Moreover, batteries have not only become cheaper but also more energy-dense. The energy density of lithium-ion cells has seen a 3.4-fold increase since 1991, meaning today’s batteries are smaller and lighter, facilitating their integration into various technologies. This improvement is vital for the electrification of sectors like aviation and heavy transportation, where the weight and size of batteries have traditionally been prohibitive.
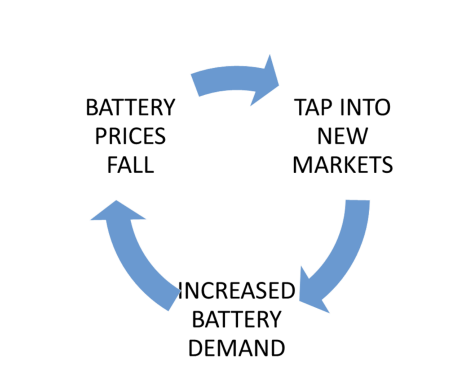
In essence, economies of scale have been instrumental in driving down the costs and enhancing the performance of lithium-ion batteries. While these batteries are now more affordable and compact, ongoing innovations and investments are required to continue this trend and fulfill the promise of electrifying sectors beyond personal transportation. The future of low-carbon electricity relies not only on the availability of renewable sources but also on the ability to store this energy economically.
Strong Industry Tailwinds Provided By Legislature
The United States’ legislative landscape, particularly shaped by acts like the Infrastructure Investment and Jobs Act, the CHIPS and Science Act, and the Inflation Reduction Act, is creating a dynamic and supportive environment for DLE companies. These legislations collectively channel substantial funds into clean energy, paving the way for the growth of DLE technologies and practices. The Infrastructure Investment and Jobs Act is crucial in this transformative shift. It earmarks significant funds across several initiatives that intersect directly with the DLE industry. For instance, the Battery and Critical Minerals Mining and Recycling Grant Program, with a substantial allocation of $125 million, directly supports the core activities of DLE companies. This funding is vital in enabling the mining and recycling of battery materials in areas where DLE technologies are particularly relevant and beneficial.
Furthermore, initiatives like the Earth Mapping Resources Initiative and the US Geological Survey’s Energy and Minerals Research Facility, with allocations of $320 million and $167 million, respectively, enhance foundational geological research. This research is essential for identifying and assessing lithium resources, which can benefit DLE operations in terms of efficiency and effectiveness. The Rare Earth Elements Demonstration Facility Program, receiving $140 million and significant funding allocated for Battery Materials Processing and Battery Manufacturing Recycling, totaling $2.8 billion, underscores a strategic focus on the entire lifecycle of battery components. From extraction to end-of-life processing, these programs offer DLE enterprises opportunities to integrate into broader supply chains or lead innovations in material processing and recycling.
Complementing these initiatives, the CHIPS and Science Act, passed in August 2022, focuses on reducing dependency on international sources for semiconductors, a critical component in the EV industry. This act indirectly supports the DLE sector by fostering semiconductor research and advanced manufacturing. The increase in demand for domestically extracted lithium, used in EV batteries, is anticipated due to this act.
Moreover, the Inflation Reduction Act, passed in the same month, accelerates momentum towards clean energy initiatives and domestic manufacturing. This act extends and expands credits for advanced energy projects and establishes new credits for advanced manufacturing production. These measures could significantly facilitate the development and scaling of DLE technologies. Additionally, the act employs the Defense Production Act to boost material security and expand loan programs for advanced technology vehicle manufacturing, which could lead to an increased demand for lithium.
A critical element of the Inflation Reduction Act is the Clean Vehicle Tax Credit, which offers up to $7,500 for consumers purchasing new qualified EVs. Alongside the mandate that critical minerals be sourced domestically or from free trade partners, this provision is particularly favorable for DLE companies. It suggests an accelerated transition to domestically sourced lithium, bolstering the market for companies capable of meeting this burgeoning demand.
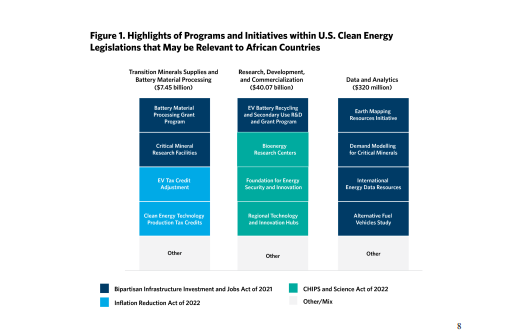
These legislative measures inject capital into the industry and shape a robust ecosystem that supports sustainable lithium extraction, processing, recycling, and end-use applications. This represents a horizon of opportunity for DLE companies, positioning them at the forefront of sustainable extraction technology amidst increasing demand for domestically sourced battery materials.
2023, from https://carnegieendowment.org/2023/10/02/how-can-african-countries-participate-in-u-s-clean-energy-supply-chains-pub-90673
Risks
The landscape of DLE technology is fraught with numerous challenges and risks that span technological, environmental, and market dynamics. DLE’s primary technological challenge is scaling up the extraction process efficiently. The NREL highlights the need for materials capable of selectively bonding with lithium ions within a milieu of other minerals and metals in geothermal brines. Achieving this requires materials that exhibit an extraordinarily high affinity for lithium ions while simultaneously rejecting other ions present in the brine. Developing such materials demands significant advancements in materials science, particularly in chemistry and nanotechnology. These materials must be durable, cost-effective, and efficient over repeated usage cycles to make the DLE process commercially viable. The challenge intensifies when considering the variability in brine composition across different lithium sources, which may necessitate custom solutions for each extraction site.
Post-extraction, the spent brine – now devoid of lithium but containing all other elements – poses a significant environmental and operational challenge. When reinjected into the salar, this spent brine can dilute the concentration of lithium in the remaining brine. Over time, this dilution could progressively lower the lithium concentration, making extraction increasingly inefficient and costly. Addressing this issue requires innovative solutions in brine management. One potential approach is developing methods to replenish the extracted lithium or separate the spent brine to prevent it from affecting the salar’s overall lithium concentration. T
This could involve complex hydrological and geological interventions to ensure that the re-injected brine does not interfere with ongoing extraction processes. From a market perspective, DLE’s viability is inextricably linked to the EV market and lithium pricing. A decline in EV sales or a drop in lithium prices can adversely impact the demand for lithium, thereby influencing the economic feasibility of DLE projects. This connection necessitates a keen understanding of the global EV market trends and factors influencing lithium pricing, such as production costs, geopolitical developments, and advances in battery technology. Additionally, the potential risk of an oversupply of lithium raw materials could create market saturation, leading to price volatility. This necessitates a strategic approach from DLE companies, including market forecasting and developing contingency plans to navigate periods of oversupply or reduced demand.
Furthermore, there’s a potential concern regarding the oversupply of lithium raw material units. While an oversupply might not immediately impact the availability of battery-grade lithium units needed for EVs and other technologies, it could lead to market saturation and price fluctuations. Such a scenario would need careful market analysis and strategic planning from companies involved in DLE to navigate these fluctuations effectively.
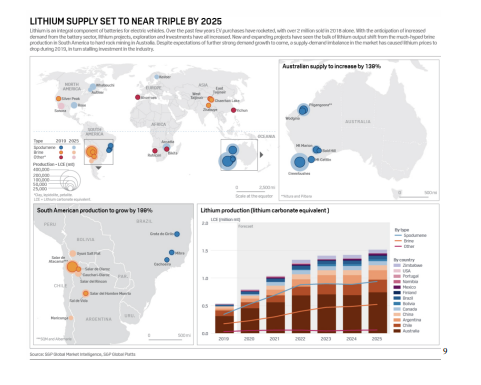
9 Latham, E., & Kilbey, B. (n.d.). Lithium supply is set to triple by 2025: Will it be enough? S&P Global. https://www.spglobal.com/en/research-insights/articles/lithium-supply-is-set-to-triple-by-2025-will-it-be-eno ugh
While DLE presents a revolutionary approach to lithium extraction with its potential for high efficiency and lower environmental impact, it faces significant challenges. These include the need for advanced material development for selective lithium ion bonding, the potential for dilution of lithium concentration in brines, and sensitivity to market fluctuations in EV sales and lithium pricing. Addressing these challenges requires a multifaceted approach encompassing technological innovation, careful resource management, and market analysis
Next Steps:
Each level of the lithium value chain demands specific actions. The advancement of new technologies, particularly DLE, is essential. DLE can potentially enhance lithium production from conventional brines by increasing recovery levels. More importantly, it can unlock lithium from inaccessible sources, such as geothermal or oilfield brines. However, this necessitates substantial investment in research and development to refine and improve these technologies, making them more efficient and environmentally sustainable.
Exploration of new lithium sources is another critical area. As of 2021, nearly 90 percent of lithium mining was concentrated in just three countries: Australia, Chile, and China. This geographical concentration presents risks regarding supply chain resilience and market control. Expanding exploration efforts into other regions helps diversify the resource base, reducing reliance on a few key locations and uncovering new, significant sources of lithium. This geographical diversification is a strategic move to ensure a steady supply of lithium and a necessary step in mitigating geopolitical risks that could disrupt the lithium market.
Clear communication and early warning from manufacturers regarding their lithium requirements are also vital. End users such as original equipment manufacturers (OEMs) and entities involved in computer-aided manufacturing play a crucial role. By signaling their product specifications and required lithium volumes well in advance, they can provide lithium miners with sufficient lead time to adapt their production processes and capacities accordingly. This proactive communication can help align the supply chain with market demands, ensuring that lithium production is in step with the evolving needs of the EV and battery industries.
The path to adequately meeting the world’s lithium needs is collaborative innovation, strategic exploration, and proactive market alignment. This pathway secures the necessary resources for the EV revolution and heralds a new era of sustainability and efficiency in lithium production and usage. At this pivotal juncture, the actions taken today will shape the future of energy, transportation, and environmental stewardship.